Introduction
In the vast realm of optics, the study of light and its interactions with various materials has always been a subject of intrigue. While linear optics deals with the behavior of light in materials where the response is directly proportional to the input, nonlinear optics delves into the more complex scenarios where this proportionality breaks down. In this article, we will journey through the fascinating world of nonlinear optics, exploring how light behaves differently in nonlinear optical materials and the myriad of applications this knowledge has birthed.
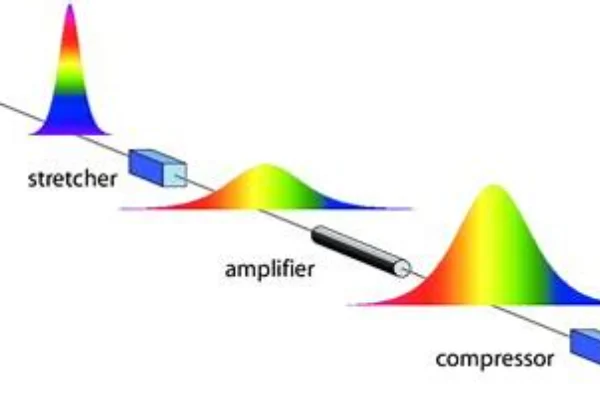
The Basics of Nonlinear Optics
The captivating world of optics has always been a subject of continuous exploration and discovery. Among its many branches, nonlinear optics stands out, offering insights into phenomena that challenge our conventional understanding of light and matter.
At its core, nonlinear optics is concerned with how light interacts with materials in ways that are not directly proportional to the light’s electric field. This means that as the intensity or properties of the incoming light change, the material’s response can change in non-intuitive and often unpredictable ways. This nonlinearity is what gives rise to a plethora of fascinating optical phenomena that are absent in linear optical systems.
For instance, imagine shining a flashlight through a clear piece of glass. In a linear optical scenario, the light would pass through the glass, perhaps getting slightly bent or refracted, but largely remaining unchanged. However, in a nonlinear optical material, that same flashlight beam might undergo dramatic changes in color, intensity, or other properties, depending on the specifics of the material and the light.
Linear optics, as the name suggests, deals with scenarios where the behavior of light is straightforward and predictable. If you double the intensity of the light source in a linear system, the response from the material (like its brightness or refractive index) would also double. Everything behaves in a straight line, with direct proportionality between cause and effect.
However, nonlinear optics throws this straightforward relationship out the window. Here, doubling the intensity of the light might more than double the material’s response, or it might have some other unexpected effect entirely. This is because the material’s response is dependent on the light’s electric field in a more complex manner. The relationship between the input (light) and the output (material’s response) is no longer a simple straight line but can be a curve, a loop, or any other shape.
This distinction between linear and nonlinear optics is not just a theoretical curiosity. It has profound implications for technology, science, and our understanding of the universe. Nonlinear optical effects are at the heart of many modern technologies, from high-speed optical communication systems to advanced medical imaging techniques. They allow us to manipulate light in ways that were once thought impossible, opening up new frontiers in research and application.
In conclusion, while linear optics provides the foundation for our understanding of light and its interactions, nonlinear optics offers a window into the more complex, intricate, and often surprising ways that light and matter can interact. It’s a field that continues to challenge and inspire, pushing the boundaries of what we know and what we can achieve with the power of light.
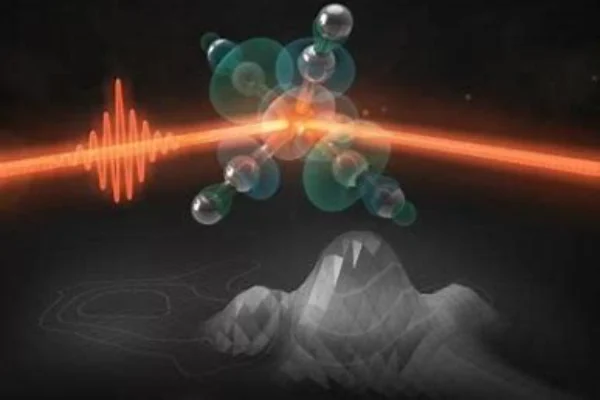
Phenomena in Nonlinear Optics
Phenomena in nonlinear optics are not only intriguing from a scientific perspective but also have significant practical applications. Let’s delve into some of the most well-known phenomena in this field.
Frequency doubling, or second harmonic generation, is a process where two photons at a given frequency combine within a nonlinear material to produce a single photon with twice the frequency (or half the wavelength) of the original photons. This phenomenon is not just a theoretical curiosity; it has real-world applications in areas such as laser technology, where it is used to change the color of laser light. For example, the green laser pointers that are commonly used in presentations often utilize frequency doubling to convert infrared laser light into green.
Parametric amplification is another fascinating phenomenon in nonlinear optics. In this process, an intense “pump” beam of light can amplify a weaker “signal” beam, all while generating a third “idler” beam. This process conserves both energy and momentum and is widely used in optical communication systems. It allows for the amplification of weak signals without the need for electronic amplification, enabling long-distance communication with less signal loss and noise.
Self-phase modulation and spectral broadening are also significant phenomena in nonlinear optics. As light travels through a nonlinear medium, the intensity of the light can alter its phase, leading to self-phase modulation. This can result in the broadening of the spectrum of a short pulse of light, which has applications in the generation of ultra-broadband light sources. This broadening effect is utilized in supercontinuum generation, where a broad spectrum of light is produced from a single laser source. It has applications in areas such as spectroscopy and biomedical imaging, where a wide range of wavelengths is required.
These phenomena illustrate the richness and complexity of nonlinear optics. They show how the nonlinear interaction of light with matter can lead to unexpected and useful outcomes, enabling technologies that were once thought impossible. From everyday devices like laser pointers to advanced communication systems and medical technologies, the phenomena of nonlinear optics continue to shape our world in profound ways.
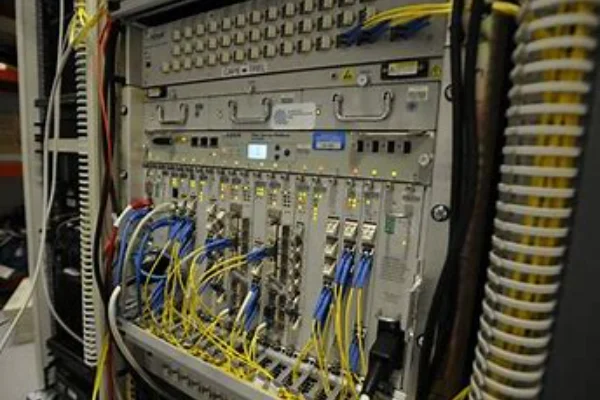
Applications in Laser Technology
Applications in laser technology have seen revolutionary advancements, thanks to the principles of nonlinear optics. These applications span a wide range of fields, from communication to medicine, and have paved the way for innovations that were once deemed futuristic.
High-intensity laser pulses are a cornerstone in many scientific and industrial applications. Nonlinear optical processes play a crucial role in generating these pulses. One notable technique is chirped pulse amplification, which garnered the 2018 Nobel Prize in Physics. This method involves stretching a short-duration laser pulse, amplifying it, and then compressing it back to its original duration. The result is an ultra-short, high-intensity laser pulse that can be used in various applications, from medical surgeries to particle acceleration.
Laser frequency conversion is another significant application. Lasers typically emit light at specific, fixed wavelengths. However, many applications require light of a different wavelength than what a given laser can provide. This is where nonlinear optics comes into play. Processes like frequency doubling can take a laser beam and convert it to a beam with half the original wavelength. Similarly, parametric down-conversion can produce two photons with longer wavelengths from a single photon. These techniques are invaluable in areas like telecommunications, where specific wavelengths are needed for data transmission.
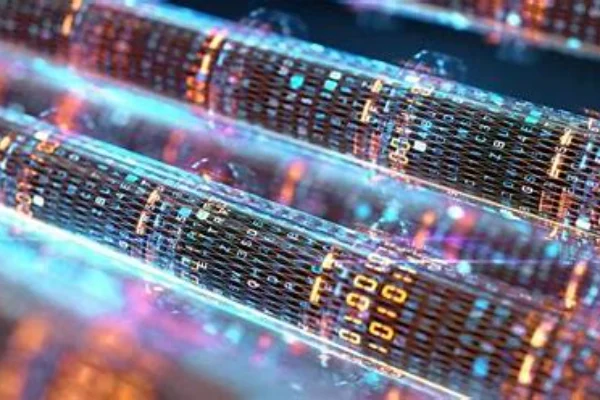
Lastly, the realm of optical switching and computing holds immense promise. Traditional electronic computers use electric currents to process and store data. However, as we approach the limits of miniaturization and speed with electronic components, there’s a growing interest in using light for computing. The nonlinear properties of certain materials can be used to create optical switches, where a light beam can control another light beam. This principle can be extended to develop optical logic gates, the building blocks of computers. The advantage of optical computing is clear: light can travel and process data much faster than electricity, offering the potential for ultra-fast, energy-efficient computing systems.
In conclusion, the principles and phenomena of nonlinear optics are not just academic curiosities. They are fundamental to many cutting-edge applications in laser technology, shaping the present and future of various industries and research fields.
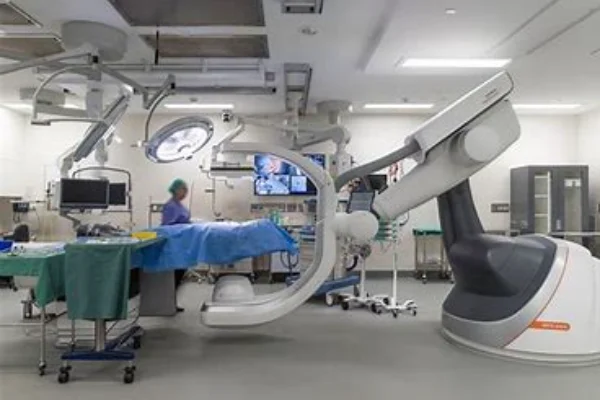
The Future of Nonlinear Optics
As we continue to push the boundaries of what’s possible with light, nonlinear optics remains at the forefront of many technological advancements. From quantum computing to medical imaging, the principles and phenomena of nonlinear optics will undoubtedly play a crucial role in shaping the future of science and technology.
Conclusion
The realm of nonlinear optics, with its rich tapestry of phenomena and applications, offers a glimpse into the vast potential of light. As we’ve explored, the behavior of light in nonlinear optical materials leads to groundbreaking applications in laser technology and beyond. As researchers continue to delve deeper into this field, there’s no doubt that the mysteries unraveled will continue to illuminate our path forward in the world of optics.
Frequently Asked Questions (FAQs)
- What causes the nonlinearity in optical materials?
- The nonlinearity arises due to the interaction of light with the electrons in a material. At high intensities, these interactions can become nonlinear, leading to the observed effects.
- How is frequency doubling different from frequency halving?
- Frequency doubling produces light with twice the frequency (or half the wavelength) of the input light. Frequency halving would produce light with half the frequency (or double the wavelength) of the input, though it’s less commonly discussed in the context of nonlinear optics.
- Can nonlinear optics be observed in everyday life?
- While the effects of nonlinear optics are typically observed in laboratory settings, some phenomena, like the green color of certain laser pointers, are a result of frequency doubling from infrared to green light.
- Are there any limitations to using nonlinear optical processes?
- Yes, the efficiency of nonlinear processes can be low, and they often require high-intensity light sources. Additionally, the materials used can be damaged by high-intensity light.
- What are the safety concerns when working with nonlinear optical processes?
- Due to the high intensities involved, there’s a risk of eye or skin damage. Proper safety equipment, like goggles and protective clothing, is essential when working with high-intensity lasers and nonlinear materials.