In the vast world of optics and photonics, the study of stimulated emission in crystals and its role in laser amplification stands as a cornerstone. The ability of certain materials, particularly crystals, to absorb and subsequently emit light under specific conditions has paved the way for the development of lasers, which have revolutionized numerous fields from medicine to telecommunications. This article delves deep into the absorption and emission properties of crystals, guiding readers through the intricate dance of photons within these materials and shedding light on the mechanisms that underpin laser amplification.
Absorption and Emission: The Basics
Understanding the fundamental processes of absorption and emission of light in crystals is crucial for various scientific and technological applications. Let’s delve into the basics of these processes and explore their significance.
Absorption in Crystals
When light interacts with a crystal, it undergoes a process known as absorption. During absorption, photons from the incident light are absorbed by the crystal, leading to the elevation of electrons from a lower energy state to a higher one. The energy required for this transition corresponds to the energy of the absorbed photons. The specific wavelengths or frequencies of light that a crystal can absorb are determined by its band structure, which is a fundamental property of the material.
Absorption is often visualized as peaks in an absorption spectrum, where the y-axis represents the intensity of absorbed light, and the x-axis corresponds to the wavelength or frequency of light. These absorption peaks provide valuable information about a crystal’s optical properties and are essential for applications like spectroscopy and photodetectors.
Understanding a crystal’s absorption characteristics is particularly important in fields like materials science and solid-state physics. It allows researchers to select appropriate materials for specific optical devices and tailor their properties to suit desired applications.
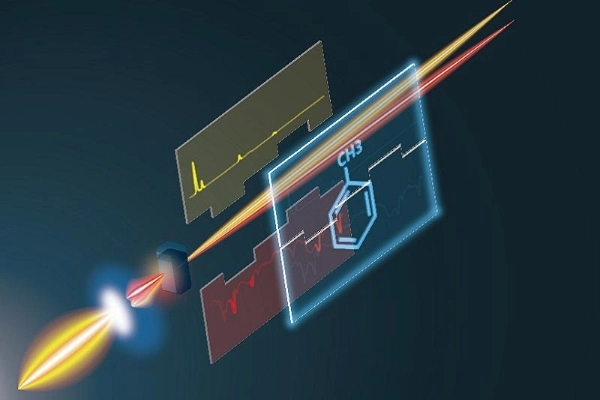
Emission: The Release of Energy
Once an electron in a crystal is excited to a higher energy state through absorption, it does not remain in that state indefinitely. After a certain period, the excited electron undergoes a transition back to its original, lower energy state. During this process, the excess energy acquired during absorption is released in the form of a photon. This phenomenon is known as emission.
The emitted photon typically carries the same energy as the absorbed one, resulting in a photon with the same wavelength or frequency. This is referred to as spontaneous emission and is a fundamental process in many natural phenomena, including fluorescence and phosphorescence.
However, it’s important to note that in some cases, non-radiative processes can occur during the electron’s return to the ground state. These non-radiative processes can lead to the emission of photons with different wavelengths or even the dissipation of energy as heat.
Emission processes are central to various technologies, including light-emitting diodes (LEDs) and lasers. In LEDs, the controlled emission of photons is used to produce visible light, while in lasers, a specific type of emission called stimulated emission is harnessed to achieve coherent and highly focused light amplification.
Stimulated Emission: The Heart of Lasers
Stimulated emission is a remarkable process at the heart of laser operation. It occurs when an already excited electron is triggered to emit its photon prematurely by encountering another photon with the same energy. This interaction results in the simultaneous emission of two identical photons, which are coherent in both phase and direction.
Stimulated emission is the key principle behind laser technology. In a laser, a medium (often a crystal) is pumped with energy to create a population inversion, where more electrons are in excited states than in the ground state. When photons encounter these excited electrons, they stimulate the emission of additional photons with the same properties. These photons bounce back and forth between mirrors in a laser cavity, leading to the coherent and amplified emission of laser light through one of the mirrors.
Lasers find extensive use in a wide range of applications, including cutting-edge research, medical procedures, telecommunications, and manufacturing. The precise control and intense brightness of laser light make it an indispensable tool in modern science and technology.
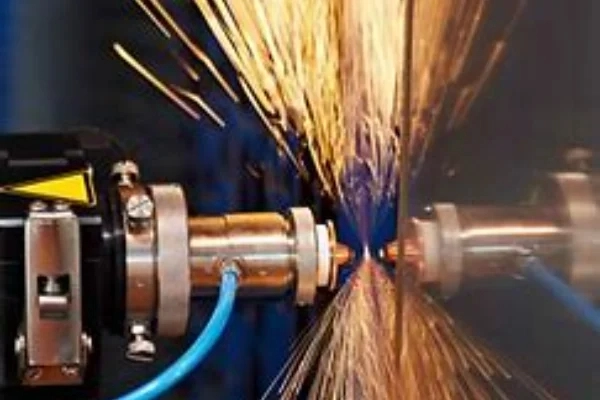
Regulating Absorption and Emission Peaks
Controlling the absorption and emission peaks in crystals is essential for tailoring their optical properties to specific applications. Several factors play a crucial role in achieving this control.
The Role of Doping
Doping is a well-established technique for regulating the absorption and emission peaks in crystals. It involves intentionally introducing impurities or dopants into the crystal lattice. These dopants alter the electronic structure of the crystal, leading to shifts in its absorption and emission spectra.
For example, doping a crystal with specific transition metal ions can create energy levels within the crystal’s bandgap, enabling the absorption and emission of light at specific wavelengths. This is widely used in laser technology and the production of laser gain media. Rare earth ions, such as neodymium (Nd) or erbium (Er), are commonly doped into laser crystals to achieve specific emission wavelengths suitable for various applications.
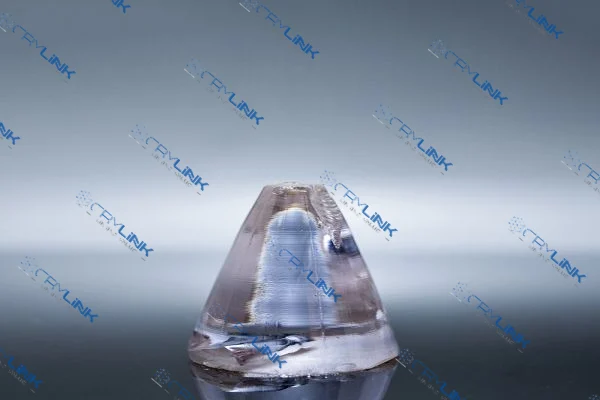
Temperature and Pressure Effects
Temperature and pressure are external factors that can significantly influence a crystal’s optical properties. By varying these parameters, researchers can fine-tune the positions of absorption and emission peaks.
Temperature-dependent shifts in absorption and emission peaks are commonly observed in semiconductor materials. This phenomenon, known as the temperature dependence of bandgap energy, allows for precise control of the emitted light’s wavelength. In high-pressure environments, crystals can undergo structural changes that impact their electronic band structure, leading to alterations in absorption and emission properties.
The ability to manipulate temperature and pressure provides flexibility in designing crystal-based optical devices for specific operating conditions and applications.
Crystal Morphology and Size
The physical characteristics of a crystal, including its morphology and size, can influence its optical properties. For instance, nano-sized crystals exhibit quantum confinement effects, which result from the confinement of charge carriers within a small volume. These effects lead to shifts in absorption and emission spectra.
In nanostructured crystals, the electronic energy levels become discrete due to quantum confinement. As a result, the crystal’s absorption and emission peaks may shift to higher energies, producing blue-shifted spectra. This phenomenon is exploited in nanomaterials for applications like quantum dots used in displays and biological imaging.
Laser Amplification through Stimulated Emission
Laser amplification is a cornerstone of laser technology and relies on the principle of stimulated emission. In a laser medium, such as a crystal, a population inversion is created, where more electrons are in excited states than in ground states. When these excited electrons undergo stimulated emission, they release photons with the same energy, phase, and direction as the incident photons.
The process of stimulated emission leads to a cascade of identical photons, resulting in coherent and amplified laser light. This intense, directional beam of light is the hallmark of laser technology, and it has revolutionized numerous fields, including telecommunications, materials processing, and medical applications.
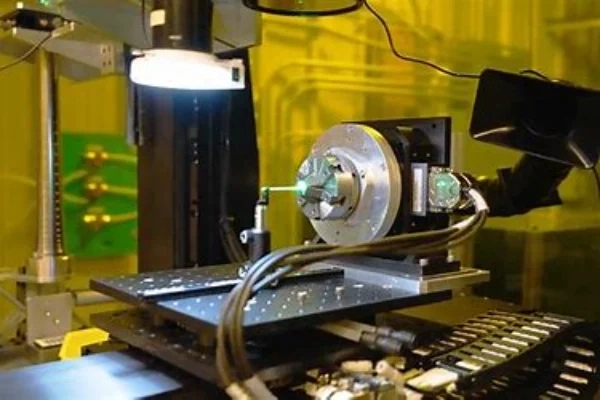
The Future of Laser Technology
As materials science and photonics continue to advance, the potential applications of stimulated emission in crystals are expanding. The future of laser technology holds exciting possibilities across various domains.
In quantum computing, the precise control of emission wavelengths using doped crystals could enable the development of quantum bits (qubits) and quantum gates, advancing the field of quantum information processing.
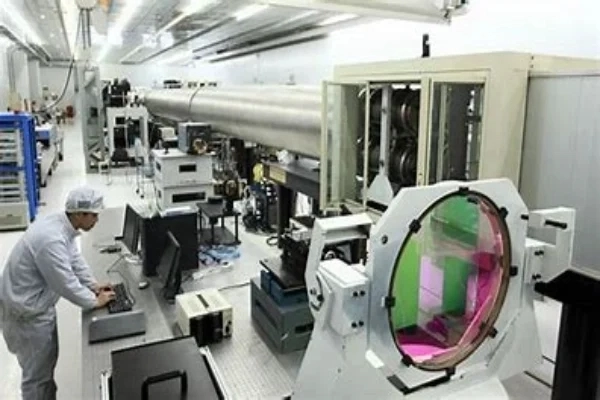
In medical imaging, laser technology utilizing crystals may offer enhanced capabilities for early disease detection and treatment. Crystals with tailored emission spectra could improve the sensitivity and specificity of imaging techniques like fluorescence microscopy.
In summary, the ability to regulate absorption and emission peaks in crystals through techniques such as doping, temperature and pressure control, and nanostructuring opens doors to a wide range of applications. Laser technology, driven by stimulated emission in crystals, is poised to play a pivotal role in the future, with innovations spanning quantum computing, medical imaging, telecommunications, and beyond.
Conclusion
The journey from understanding the basic absorption and emission properties of crystals to harnessing the power of stimulated emission for laser amplification is a testament to the marvels of science and engineering. As we continue to push the boundaries of what’s possible, crystals, with their unique optical properties, will undoubtedly remain at the forefront of technological advancements, lighting the way for future innovations.
FAQs
- What is the difference between spontaneous and stimulated emission?
- Spontaneous emission is the natural process where an excited electron returns to its ground state, emitting a photon. Stimulated emission, on the other hand, occurs when an external photon forces an excited electron to emit its photon earlier than it would have naturally.
- Why are certain crystals preferred in laser technology?
- Certain crystals offer a combination of desirable properties such as high transparency, suitable absorption and emission peaks, and the ability to sustain a population inversion, making them ideal for laser applications.
- How does doping affect a crystal’s optical properties?
- Doping introduces impurities into a crystal, which can create new energy levels or modify existing ones. This can lead to shifts in absorption and emission spectra, making it possible to tailor a crystal’s optical properties for specific applications.
- What is population inversion in the context of lasers?
- Population inversion refers to a condition where more electrons in a material are in excited states than in ground states. This is a prerequisite for achieving laser action through stimulated emission.
- Are all crystals suitable for laser applications?
- No, not all crystals are suitable for laser applications. The choice of crystal depends on several factors, including its optical properties, thermal stability, and ability to sustain a population inversion.