Introduction to Ti:Sapphire Laser Systems
Ti:Sapphire Oscillators and Amplifiers represent breakthroughs in the field of laser technology. Utilizing Titanium:Sapphire mediums, these devices showcase exceptional thermal properties, broad gain bandwidth, and high optical damage thresholds. This enables the generation of ultrashort, high energy pulses, redefining the boundaries of laser technology.
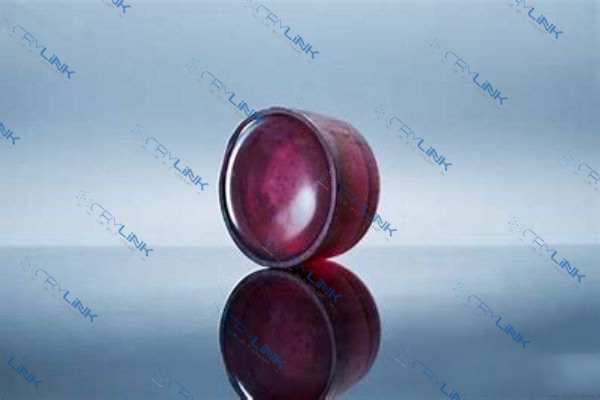
Exploring Advanced Techniques and Considerations Using Ti:Sapphire Oscillators and Amplifiers
The prowess of Ti:Sapphire Oscillators and Amplifiers lies in their adaptability and proficiency in employing advanced techniques, specifically Chirped Pulse Amplification (CPA) and Optical Parametric Amplification (OPA). Understanding these techniques and their associated considerations is crucial to fully exploit the potential of Ti:Sapphire based systems.
At the forefront of laser technology, Chirped Pulse Amplification (CPA) stands out as an extraordinary technique revolutionizing our capacity to generate high-energy, ultrashort laser pulses. Born out of a necessity to circumvent the power constraints of laser amplifiers, CPA ingeniously leverages the concept of ‘chirping’ – modulating the frequency of a laser pulse with time – to significantly enhance its energy without risking damage to the amplifier.
The first stage of the CPA process involves stretching the initial ultrashort pulse. This is achieved using a pair of diffraction gratings or a specially designed optical fiber. As the pulse travels through these media, its spectral components are spread out in time due to dispersive effects, resulting in a ‘chirped’ pulse of longer duration and thus lower peak power. This stretching process is meticulously calibrated to ensure that, once amplified and recompressed, the pulse can be restored to its original ultrashort duration.
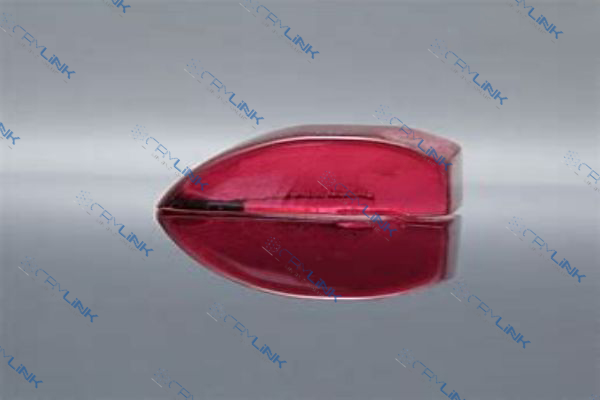
The second stage of CPA sees the stretched pulse enter the amplifier. Since the pulse has been elongated, its peak power is substantially reduced, enabling the amplifier to boost the pulse energy without reaching its damage threshold. This feature of CPA is particularly valuable, as it allows us to harness the high gain of amplifiers like those based on Ti:Sapphire, which can amplify the pulse energy by several orders of magnitude.
Following amplification, the final stage of CPA involves recompressing the pulse to its original ultrashort duration. This is typically achieved using another pair of diffraction gratings arranged in a configuration opposite to that used for stretching. The recompression process reverses the chirp introduced during the stretching stage, effectively concentrating the energy of the pulse in time. The result is a laser pulse of immense peak power, far exceeding what could have been achieved by directly amplifying the initial ultrashort pulse.
As with any advanced technique, mastering CPA requires a nuanced understanding of its intricacies. Careful management of the spectral phase during the stretching and recompression stages is critical to ensure efficient amplification and to maintain the quality of the pulse. Despite these complexities, the benefits reaped by implementing CPA – namely the ability to produce ultrashort, high-energy pulses – are well worth the effort. Indeed, the technique’s versatility has seen it deployed in diverse applications, from precision materials processing and medical surgery to cutting-edge scientific research.
In summary, CPA represents a watershed moment in laser technology, elegantly solving the problem of amplifier damage while pushing the boundaries of what we can achieve with lasers. Its mastery is a testament to our ingenuity, our desire to overcome obstacles, and our relentless pursuit of innovation.
The other notable technique, Optical Parametric Amplification, manipulates the concept of energy conservation to produce broad bandwidth amplification. In OPA, a high-energy pump beam is divided into two beams: a signal and an idler. While the signal is amplified, the idler aids in maintaining energy conservation. Considerations in OPA include the phase-matching condition and the temporal overlap of the interacting pulses, which can influence the efficiency of the amplification and the spectral characteristics of the output.
Both CPA and OPA techniques significantly contribute to the generation of ultrashort pulses, the broadening of the spectrum, and the achievement of high energy outputs. Their advanced capabilities make them well-suited for scientific and industrial applications requiring precise, high-energy, and ultrashort laser pulses.
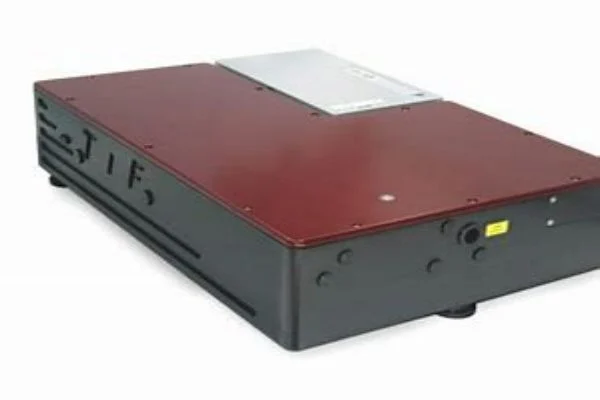
By adopting these advanced techniques, Ti:Sapphire Oscillators and Amplifiers are becoming crucial in pushing the boundaries of several research areas. These include attosecond science, which studies ultrafast electron dynamics, high-harmonic generation, which allows frequency multiplication, and laser-driven particle acceleration, opening new doors in physics and materials science. As we continue to unravel and refine these techniques, we can expect even more ground-breaking advancements in the future.
Benefits of Advanced Techniques in Laser Technology
The advanced techniques employed by Ti:Sapphire Oscillators and Amplifiers – notably Chirped Pulse Amplification (CPA) and Optical Parametric Amplification (OPA) – come with a wealth of benefits. These techniques are pivotal in the generation of ultrashort pulses, broadening the spectrum, and achieving high energy outputs, facilitating breakthroughs in various scientific and industrial applications.
The most significant contribution of CPA and OPA techniques is their capacity to generate ultrashort pulses. These ultrashort pulses, in the order of femtoseconds (10^-15 seconds), are integral to the study of ultrafast phenomena such as electron dynamics and chemical reactions. With CPA, the stretched laser pulse can be amplified to high energies and then recompressed to ultrashort durations, providing massive peak powers. Simultaneously, OPA can convert a portion of a pump beam into a lower-energy signal beam, which can then be tailored to have ultrashort pulse durations.
The capability to broaden the spectrum of laser pulses is another significant advantage. Broad bandwidths enable the production of ultrashort pulses, a requirement for many applications. In CPA, the spectrum of the pulse can be broadened using self-phase modulation in a highly nonlinear medium, which helps in stretching the pulse before amplification. OPA, on the other hand, allows for a broad gain bandwidth that is tunable across a wide spectral range, providing flexible control over the central wavelength of the amplified signal.
The quest for higher energy outputs is never-ending in laser technology. CPA is a particularly effective technique for achieving this, as it allows for the amplification of pulses to high energies without causing damage to the amplifier, thanks to the initial stretching of the pulse. OPA also contributes to this end; it is capable of supporting high energy conversion efficiencies, enabling significant amplification of the signal beam.
In conclusion, these advanced techniques have ushered in a new era in laser technology. By generating ultrashort pulses, broadening the spectral range, and enabling high energy outputs, they have significantly expanded our ability to probe and manipulate matter, leading to unprecedented discoveries and applications.
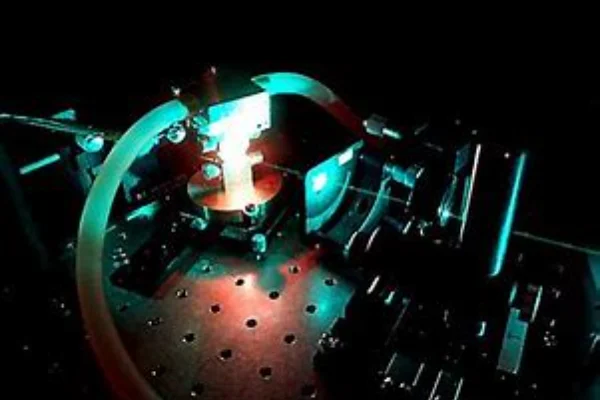
Cutting-Edge Applications Enabled by Ti:Sapphire-Based Systems
Ti:Sapphire-based systems, armed with advanced techniques like CPA and OPA, are at the heart of some groundbreaking applications. From probing the frontiers of attosecond science to driving novel particle acceleration methods, the versatility of these systems is profoundly influencing our understanding of the world.
Attosecond science, which delves into the world of ultrafast phenomena, is one such field that’s significantly benefitted from Ti:Sapphire systems. Attosecond pulses (1 attosecond = 10^-18 seconds) are essentially the fastest events created and measured by humans. They have become a powerful tool to investigate electron dynamics on their natural time scale, offering unprecedented insights into quantum mechanics and potentially paving the way for advancements in areas like photovoltaics and quantum computing. Ti:Sapphire-based systems, through their ability to generate stable, high-energy, ultrashort pulses, have become the cornerstone of attosecond pulse generation.
High-harmonic generation (HHG) is another frontier where Ti:Sapphire systems have proven instrumental. HHG is a nonlinear process where a strong laser field drives an electron to oscillate and emit radiation that is harmonically related to the driving laser. It is a versatile tool for generating coherent radiation in the extreme ultraviolet (EUV) and soft x-ray regions, which are difficult to access with traditional light sources. The high peak power and ultrashort pulse duration achievable with Ti:Sapphire amplifiers make them the ideal choice for driving the HHG process.
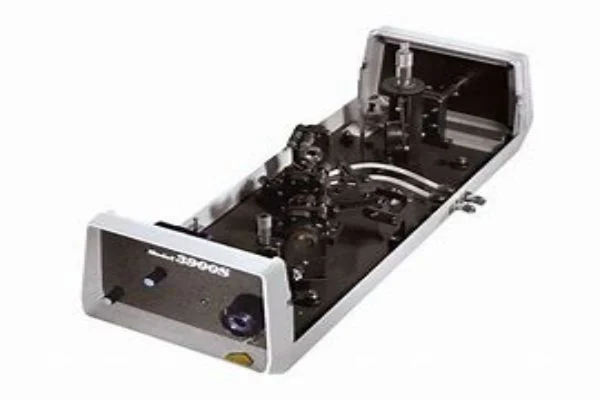
Ti:Sapphire systems are also pushing the boundaries in laser-driven particle acceleration. In this revolutionary technique, laser pulses are used to generate and drive waves in a plasma, which can then accelerate charged particles to high energies over short distances. Known as Laser Wakefield Acceleration (LWFA), this technique holds the promise for developing compact particle accelerators for various applications, including medical therapies and high-energy physics research. The high energy and short duration of laser pulses from Ti:Sapphire amplifiers make them perfectly suited for driving the LWFA process.
In sum, the cutting-edge applications enabled by Ti:Sapphire-based systems are transforming our capabilities in several scientific and technological domains. The future will undoubtedly see these systems playing an even more central role in our quest to understand and manipulate the world on increasingly smaller and faster scales.
Conclusion
Ti:Sapphire Oscillators and Amplifiers are reshaping the landscape of laser technology, providing pathways to explore uncharted territories in scientific research. Through innovative techniques such as CPA and OPA, these systems generate ultrashort pulses, broaden spectra, and achieve high energy outputs. Whether it’s attosecond science, high-harmonic generation, or laser-driven particle acceleration, the Ti:Sapphire laser systems are proving to be an invaluable asset, revolutionizing fields and promising exciting advancements in the future.
Frequently Asked Questions
- 1. What are the primary advantages of Ti:Sapphire laser systems?
- The primary advantages include the ability to generate ultrashort pulses, spectral broadening, and achieving high energy outputs.
- 2. How does Chirped Pulse Amplification (CPA) work?
- CPA works by ‘chirping’ or stretching the pulse before amplification to reduce its peak power, followed by recompression after amplification.
- 3. What does Optical Parametric Amplification (OPA) do?
- OPA splits a high-energy pump beam into lower energy signal and idler beams, enabling broad bandwidth amplification.
- 4. What applications are enabled by Ti:Sapphire laser systems?
- Applications include attosecond science, high-harmonic generation, and laser-driven particle acceleration.
- 5. How do Ti:Sapphire laser systems contribute to high-harmonic generation?
- By directing intense femtosecond pulses into a gas medium, these systems can generate high-order harmonics, extending the frequency range into the X-ray region.