Introduction to Scintillator Crystals
Scintillator crystals are a remarkable class of materials that have the ability to convert high-energy radiation into visible light. When these crystals are struck by particles or photons, they emit light, a phenomenon known as scintillation. Among the vast variety of scintillator crystals available, BGO, LYSO, and NaI(Tl) stand out for their exceptional characteristics and applications in radiation detection.
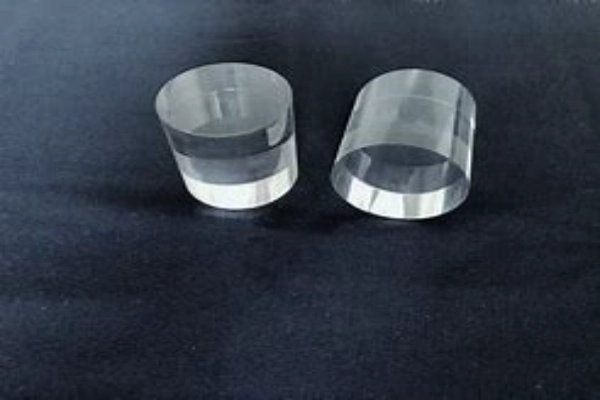
Bismuth Germanate (BGO) Crystals
Bismuth Germanate, or BGO as it is popularly known, has steadily emerged as one of the most sought-after scintillator crystals in the realm of radiation detection. The essence of BGO’s capability can be understood from its dense nature and high atomic number, which together contribute to its impressive stopping power. This essentially means that BGO is adept at halting the movement of high-energy gamma rays, capturing them efficiently. This property is not just theoretical; it translates to practical applications where the precision and efficacy of radiation detection matter the most.
Furthermore, another admirable characteristic of BGO is its intrinsic resilience against radiation damage. Many scintillator crystals, when exposed to continual radiation, tend to degrade over time, either diminishing in their efficiency or becoming altogether non-functional. However, BGO stands out in this aspect. Its inherent resistance ensures that it can function optimally for extended periods, even under conditions of consistent radiation exposure. This longevity not only makes it a cost-effective choice but also a reliable one for critical applications where downtime or frequent replacements can be detrimental.
In the practical world, the advantages of BGO are most prominently seen in its applications, especially in the medical field. Positron emission tomography, more commonly known as PET scanning, is a sophisticated imaging technique that relies heavily on the detection of high-energy photons. Given the critical nature of medical diagnoses, there is no room for error. BGO crystals, with their high density and atomic number, are perfectly suited for this task. They effectively capture these photons, ensuring that the imaging is both accurate and detailed.
Beyond the confines of medical imaging, BGO’s prowess extends to the domain of high-energy physics experiments. These experiments, often conducted in advanced research facilities, require materials that can detect and measure gamma rays with precision. BGO fits this requirement seamlessly. Additionally, in the field of gamma-ray spectrometry, where the identification and measurement of gamma rays are vital, BGO has proven to be an invaluable asset.
In conclusion, the prominence of BGO in the world of radiation detection is not merely by chance. Its unique characteristics, combined with its practical applications, make it an indispensable tool in both medical and research fields. Whether it’s aiding in accurate medical diagnoses or facilitating groundbreaking research in physics, BGO’s contribution is undeniable.
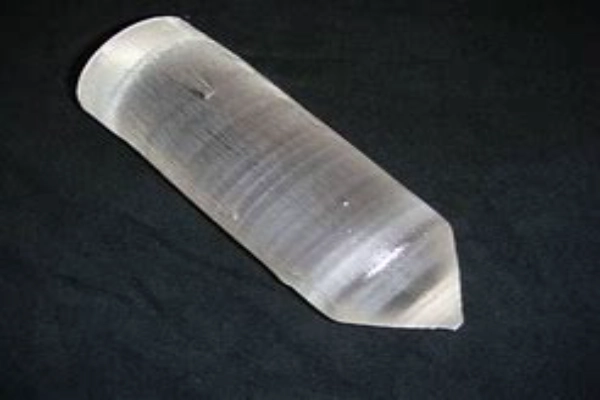
Lutetium-Yttrium Orthosilicate (LYSO) Crystals
Lutetium-Yttrium Orthosilicate, popularly known as LYSO, is a composite crystal that has garnered immense attention in the world of radiation detection. At its core, LYSO is formed by combining lutetium and yttrium orthosilicate, two elements that endow it with remarkable properties. Among these, the crystal’s ability to produce an intense light output and its rapid decay times are particularly noteworthy. These characteristics aren’t just numerically impressive but have practical implications that translate to real-world efficiency.
The science behind the efficiency of LYSO lies in its energy resolution and timing accuracy. Energy resolution is crucial in any scintillator’s ability to differentiate between closely spaced energy levels. A higher energy resolution means that the scintillator can detect and distinguish between different energies more accurately.
LYSO, with its excellent light output, excels in this aspect, ensuring that every particle interaction is captured with precision. Coupled with this is the fast decay time, which essentially refers to how quickly the scintillator returns to its ground state after emitting light. A faster decay time means the scintillator is ready to detect subsequent particles more quickly, leading to more accurate timing measurements. Together, these attributes make LYSO a powerhouse in terms of both energy resolution and timing accuracy.
When we delve into the practical applications of LYSO, its prominence in medical imaging becomes evident. The realm of medical imaging, especially techniques like PET scanning, demands absolute precision. Even the slightest errors can lead to misdiagnoses, with potentially grave consequences. LYSO’s rapid response time is a boon in this context. It ensures that the images produced are sharp, capturing even the minutest details. Furthermore, the high light yield of LYSO plays a pivotal role in detecting even the tiniest amounts of radiation. In medical terms, this translates to more accurate diagnoses, ensuring that ailments are identified correctly and treated appropriately.
In essence, LYSO isn’t just another scintillator crystal. Its unique combination of properties, from its composition to its performance metrics, makes it a frontrunner in radiation detection. Whether it’s aiding doctors in making accurate medical decisions or facilitating cutting-edge research, LYSO’s significance in the modern world is truly unparalleled.
-crystals.webp)
Sodium Iodide (NaI(Tl)) Crystals
Sodium Iodide crystals, when doped with thallium, yield the unique and versatile NaI(Tl) scintillator, a mainstay in the realm of radiation detection. The fundamental allure of NaI(Tl) is rooted in its exceptional light yield. In the intricate dance of photons and particles, NaI(Tl) stands out as a beacon, ensuring that every interaction is not only captured but also transformed into discernible information.
One of the standout features of NaI(Tl) is its luminescence, which peaks at a wavelength that seamlessly aligns with the operational spectrum of photomultiplier tubes. This alignment is more than just serendipitous; it’s crucial. Photomultiplier tubes play a pivotal role in amplifying the light signals generated by scintillators.
When the peak luminescence of a scintillator aligns with the photomultiplier’s sensitivity, the result is an optimal conversion of radiation into electronic signals. This synergy between NaI(Tl) and photomultiplier tubes is the cornerstone of its efficiency, ensuring that the radiation is not just detected but also accurately measured and represented.
In terms of practicality, NaI(Tl) finds itself at the heart of numerous applications, reflecting its versatility and efficiency. In the medical realm, its role is indispensable. Gamma cameras, which provide vital insights into the human body, rely on NaI(Tl) to visualize organs and tissues. The high light yield of NaI(Tl) ensures detailed and clear images, which are crucial for accurate diagnoses and subsequent treatments.
Beyond the corridors of hospitals and clinics, NaI(Tl) plays a sentinel role in industries and environmental setups. Whether it’s monitoring radiation levels in nuclear facilities or tracking radioactive materials in various environmental contexts, NaI(Tl) detectors stand vigilant, ensuring safety and compliance.
In summary, the brilliance of NaI(Tl) is not just in its luminescence but in its adaptability and efficiency. From ensuring medical accuracy to safeguarding our environment, its contributions are both diverse and profound, making it an unsung hero in the vast landscape of radiation detection.
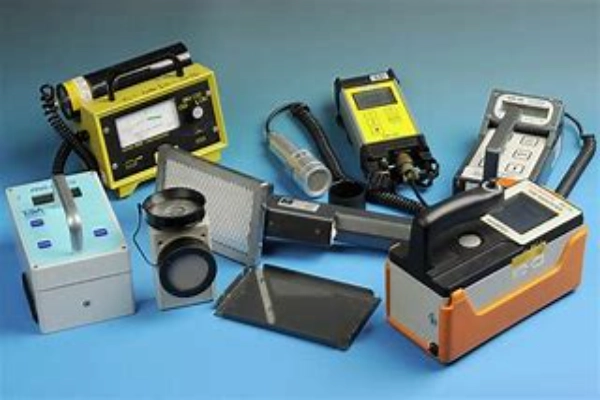
The Luminescence Mechanism Behind Scintillator Crystals
The phenomenon of luminescence in scintillator crystals is a captivating interplay of energy and matter. At its core, when ionizing radiation—be it in the form of high-energy particles or photons—interacts with a scintillator crystal, it imparts energy to the atoms within. This energy excites the atoms, pushing them to a higher energy state. However, atoms inherently prefer stability, and this excited state is transient. As they revert to their stable, ground state, the excess energy is emitted as visible light, a process we perceive as luminescence.
This fundamental mechanism, while consistent across scintillator crystals, manifests differently in terms of efficiency and speed depending on the crystal in question. Take, for instance, the trio of BGO, LYSO, and NaI(Tl). Each of these has carved a niche for themselves in the world of radiation detection, thanks to their distinct attributes. BGO, with its formidable density, possesses a pronounced stopping power, effectively halting high-energy particles in their tracks. Moreover, its resilience against radiation damage ensures it remains functional and efficient even in high-radiation environments.
In contrast, LYSO shines in scenarios where speed and light output are paramount. Its atoms return to their ground state incredibly swiftly, making it one of the fastest scintillators in terms of response time. This rapidity, combined with its impressive light output, makes LYSO a preferred choice in applications like medical imaging, where precision and clarity are crucial.
Lastly, NaI(Tl) is the jack-of-all-trades in the scintillator world. Its luminescence is characterized by an exceptional light yield, ensuring that even the minutest radiation interactions are captured. Furthermore, its versatility sees it being employed in a myriad of applications, from medical setups to industrial monitoring.
In essence, while the science behind luminescence in scintillator crystals is consistent, the choice between BGO, LYSO, and NaI(Tl) hinges on the nuances of their individual characteristics and the specific demands of the application they are employed in. Each crystal, with its unique strengths, caters to different facets of radiation detection, ensuring that the field remains versatile and adaptive.
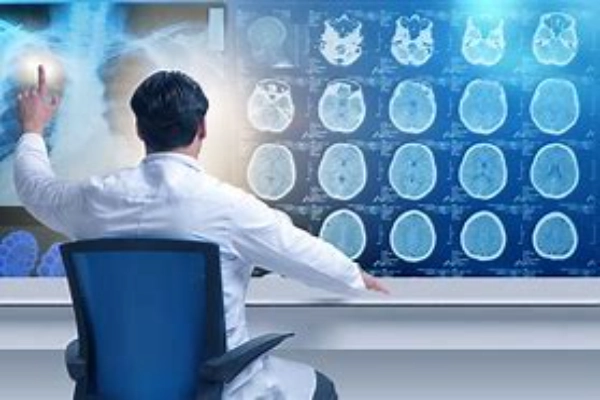
The Future of Scintillator Crystals
As technology continues to advance, the demand for more efficient and effective scintillator crystals grows. Researchers are constantly on the hunt for new materials and methods to enhance the properties of these crystals, ensuring that they remain at the forefront of radiation detection and imaging technologies.
Conclusion
BGO, LYSO, and NaI(Tl) scintillator crystals have carved a niche for themselves in the realm of radiation detection. Their unique characteristics make them indispensable in various sectors, from medical imaging to industrial applications. As we continue to push the boundaries of technology, the importance and potential of these crystals are bound to shine even brighter.
FAQs
- 1. What are scintillator crystals?
Scintillator crystals are materials that emit light when struck by particles or photons. - 2. Why is BGO preferred in PET scanners?
BGO has a high atomic number and density, making it effective in detecting the high-energy photons emitted during PET scans. - 3. How does LYSO offer sharp images in medical imaging?
LYSO’s fast response time and high light yield ensure accurate detection of radiation, resulting in clearer images. - 4. Why is NaI(Tl) used in gamma cameras?
NaI(Tl) has a high light yield and its luminescence is well-matched with photomultiplier tubes, making it efficient in converting radiation into an electronic signal. - 5. What causes the luminescence in scintillator crystals?
When ionizing radiation excites the atoms within a scintillator crystal, they release the excess energy as visible light upon returning to their ground state.